Microencapsulation of Red Sorghum Phenolic Compounds with Esterified Sorghum Starch as Encapsulant Materials by Spray Drying
Adriana García-Gurrola1
, Susana Rincón1
, Alberto A. Escobar-Puentes1
, Alejandro Zepeda2
and Fernando Martínez-Bustos3
1National Technological Institute of Mexico/I.T. Mérida, Av. Tecnológico km 4.5 S/N, C.P. 97118 Merida, Yucatan, Mexico
2Autonomous University of Yucatan, Periferico Norte kilómetro 33.5, Chuburna de Hidalgo Inn, C.P. 97203 Mérida, Yucatan, Mexico
3Center for Research and Advanced Studies of the IPN, campus Queretaro Libramiento Norponiente 2000, Fracc. Real de Juriquilla, C.P. 76230
Santiago de Queretaro, Queretaro, Mexico
Article history:
Received: 22 November 2018
Accepted: 23 May 2019
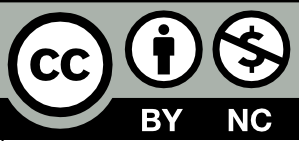
Key words:
microencapsulation, sorghum phenolic compounds, sorghum starch, esterification, extrusion
Summary:
Phenolic compounds with antioxidant properties are highly sensitive molecules, which limits their application. In response, extruded esterified starch has been proposed as efficient encapsulating material. In this work, we aim to describe the encapsulation of red sorghum phenolic compounds by spray drying using extruded phosphorylated, acetylated and double esterified sorghum starch as wall material. Their respective encapsulation yields were 77.4, 67.4 and 56.8 %, and encapsulation efficiency 91.4, 89.7 and 84.6 %. Degree of substitution confirmed esterification of the sorghum starch and Fourier transform infrared spectroscopy showed the significant chemical and structural changes in the extruded esterified starch loaded with phenolic compounds. Microcapsules from phosphorylated sorghum starch showed the highest endothermic transition (173.89 °C) and provided a greater protection of the phenolic compounds during storage at 60 °C for 35 days than the other wall materials. Extruded esterified sorghum starch proved to be effective material for the protection of phenolic compounds due to its high encapsulation efficiency and stability during storage.
*Corresponding author:
+524422119905
+524422119938
This email address is being protected from spambots. You need JavaScript enabled to view it.
INTRODUCTION
Sorghum is an important cereal grain worldwide due to its resistance to drought and its high starch content (70%); it has low production costs and multiple agroindustrial applications (1). In addition, it has been previously reported that pigmented testa of sorghum genotypes have high phenolic content (2) and hydroxybenzoic and hydroxycinnamic acids, in addition to being a unique source of 3-deoxyanthocyanidins, with high anticancer and antioxidant capacities (3). In vitro studies have shown that the exposure of cancerous cells of the colon and the oesophagus to polyphenolic sorghum extracts decreases or inhibits cell proliferation (4, 5). However, although polyphenolic phytochemicals provide various positive health benefits, some problems related to their bioactivity are a cause for major concern during food processing, since they are sensitive to oxygen, temperature, pH, light, and gastrointestinal tract environment (6). Therefore, the efficacy, bioactivity and bioavailability of phenolic compounds will depend on their preservation and stability. In this respect, the encapsulation of phenolic compounds could help to address some of the previously mentioned disadvantages. Prior studies have demonstrated that the use of encapsulated polyphenols rather than free molecules enhances stability during storage (7). To achieve this, spray drying is regarded as one of the most promising techniques for the encapsulation and protection of certain classes of phenolic compounds (8), in that this is a highly efficient, economical process, and provides chemical stability to sensitive molecules such as phenolic compounds. Additionally, this process results in quality particles, and has been employed for the preparation of dry, stable food additives and flavours (9). Several classes of phenolics, such as anthocyanins from Berberis vulgaris (10), flavonoid extract from Hypericum perforatum (11), and polyphenols from Rosmarinus officinalis L. leaves (12), have been encapsulated by spray drying to improve the storage stability of phenolic compounds.
Different biopolymers, such as protein and polysaccharides, have served for the encapsulation of phenolic compounds by spray drying. It was previously reported that the encapsulation of gallic acid by spray drying with conventionally acetylated starch resulted in an efficiency and encapsulation yield of 62 and 74.3%, respectively (13). However, previous results demonstrated that esterified and extruded types of starch were effective encapsulation agents of hydrophobic (14) and hydrophilic (15) active ingredients with high efficiencies and encapsulation yields when spray drying was utilized.
Therefore, it is clear that sorghum grain is emerging as a potential source of polyphenolic compounds with health benefits, in addition to being a cereal with high starch content. To our knowledge, there is no published information on the esterification of white sorghum starch by reactive extrusion for evaluation as a wall material. Also to our knowledge, there are no reports regarding encapsulation of red sorghum phenolic compounds using starch derivatives as wall materials. Moreover, to date and to our knowledge, no double modification of the starch has been reported to obtain a phosphorylated and acetylated starch by extrusion. Based on the latter, we are raising the following as aims in this paper: (i) to develop three different esterified sorghum starch types by reactive extrusion, (ii) to evaluate the encapsulation of red sorghum phenolic compounds by spray drying, and (iii) to evaluate the storage stability of microencapsulated red sorghum phenolic compounds.
MATERIALS AND METHODS
Materials
A white sorghum grain cultivar ’Sinaloense’ (donated by Dr Pecina from the National Institute of Forestry, Agricultural and Livestock Research (INIFAP), Guanajuato, Mexico) was used for the isolation of starch according to the wet milling method (16). A red sorghum grain cultivar ’Pajarero’, kindly donated by INIFAP Mexico, was used for the extraction of phenolic compounds. Capsul® starch was purchased from Ingredion México S.A de C.V. (Guadalajara, Mexico), hydrochloric acid, sodium hydroxide, ethanol, potassium hydroxide, phenolphthalein, potassium bromide, gallic acid, methanol, acetic acid, Folin-Ciocalteu reagent, acetic anhydride and sodium tripolyphosphate (STPP) were acquired from Sigma-Aldrich Chemical Co., Merck (St Louis, MO, USA). All reagents were of analytical grade.
Esterification of starch
Sample preparation
Native starch was hydrolyzed with HCl according to the method of Zambrano and Camargo (17) prior to esterification by reactive extrusion.
Phosphorylation of starch
Phosphorylation of starch was carried out with STPP by reactive extrusion based on the method of Chang and Lii (18). The previously hydrolyzed starch was conditioned with STPP (4 g/100 g). Briefly, the volume of distilled water necessary to adjust the moisture content to 18% was used to dissolve STPP and 0.1 M HCl on a magnetic stirrer (model FE-310; Felisa, Guadalajara, Jalisco, Mexico) for approx. 10 min. The pH of the solution was 4.5. The prepared solution was sprayed on starch and mixed vigorously with a laboratory spatula. Before extrusion, the conditioned starch was stored for 12 h at 4 °C in plastic bags to ensure a homogeneous water distribution.
Acetylation of starch
Acetylation was carried out by the extrusion process according to the method of Mali and Grossmann (19). The volume of 2.5 mL acetic anhydride was added to 100 g of hydrolyzed starch. The hydrolyzed starch was conditioned at 18% feed moisture and the pH adjusted to 8.5–9.0 with 0.1 M NaOH as previously mentioned. The obtained starch was stored for 12 h at 4 °C to ensure a homogeneous water distribution.
Double esterification of acetylated and phosphorylated starch
For double esterification, 2 g of STPP per 100 g hydrolyzed starch and 1.42 mL of acetic anhydride per 100 g of hydrolyzed starch were added simultaneously to the starch and conditioned to 18% moisture content and pH adjusted to 4.5–5.0 with 0.1 M HCl, as previously mentioned. The conditioned starch was stored for 12 h at 4 °C to ensure homogeneous water distribution.
Extrusion process
The conditioned starch samples were processed in a single screw extruder designed and manufactured by CINVESTAV-IPN (Querétaro, Mexico), with a 20-mm inner barrel diameter. Barrel temperatures were maintained at 60, 130 and 170 °C at the feeding, transition and high-pressure extrusion zones, respectively. Screw speed was 80 rpm and feed rate was 70 g/min, while the compression ratio of the screw was 3:1. The resulting extruded samples were dried in the oven (40 °C), milled and sieved through a mesh with a 149 µm pore size. Powder samples of esterified starch were packed for subsequent characterization and microencapsulation of phenolic compounds.
Degree of substitution of phosphorylated starch
Phosphorus content and degree of substitution (DS) in phosphorylated sorghum and double esterified sorghum starch were determined by the method of Smith and Caruso (20). Three repetitions were carried out for each analysis and the mean value was reported. DS was calculated as follows:
DS=(162·w(P))/(3100–124·w(P)) /1/
where w(P) is the mass fraction of phosphorus (on dry mass basis) in the phosphorylated sorghum starch, 162 is the molar mass of the anhydroglucose unit, 3100 is the atomic mass of phosphorus multiplied by 100 and 124 is the molar mass of the phosphate substituent.
Determination of the amount of acetyl groups and degree of substitution
The amount of acetyl groups and the DS of the acetylated sorghum and double esterified sorghum starch were determined by titration, as described by Wurzburg (21). Acetylated starch (1 g) was placed in a 250-mL flask, and 50 mL of 75% ethanol in distilled water were added. The stoppered flask was covered with aluminium foil, placed in a water bath at 50 °C for 30 min and then cooled. Then, 40 mL of 0.5 M KOH were added and kept under constant stirring at 200 rpm (model FE-310; Felisa) for 72 h. After that, the excess of alkali was titrated with 0.5 M HCl, using phenolphthalein as an indicator. The original, unmodified starch served as control. The molality of acetyl groups (b/%) was calculated according to the following equation:
b(acetyl)=[(Vcontrol–Vsample)·(c(HCl)+0.043·100)/msample] /2/
where Vcontrol and Vsample are the titration volumes for the blank and sample respectively (mL), msample is the sample mass (g), and 0.043 is the milliequivalent of the group CH3–C=O. DS was calculated as follows:
DS=[162·b(acetyl)/(4300–(42·b(acetyl))] /3/
where 162 is the molar mass of the anhydroglucose unit, 4300 is the atomic mass of group CH3–C=O multiplied by 100 and 42 is the atomic mass of group CH3–C=O minus 1.
Fourier transform infrared spectroscopy
Fourier transform infrared spectroscopy (FTIR) analyses were performed to determine the structural changes in derivatized starch samples (acetylated, phosphorylated and double esterified sorghum starch) compared to native starch with a spectrometer (GX PerkinElmer; Boston, MA, USA). The microparticles of the three types of starch were prepared by finely grinding the powder with KBr (1:100 m/m). The spectrum was recorded in a range between 400 and 4000 cm–1.
Polyphenolic extract
Phenolic compounds were extracted from the red sorghum grain. A sample of finely ground sorghum grain (6.25 g) was stirred in 250 mL of acidified ethanol (0.01% (V/V) HCl in 70% ethanol) (pH=2.5). After 1 h of extraction, the solution was centrifuged (Hermle Z513 K equipment; Wehingen, Germany) for 10 min at 5000×g and filtered by vacuum filtration through a Whatman No. 1 filter paper to recover the solids. The process was repeated on the solids (a 250-mL volumetric flask containing 10 mL of acidified ethanol) and the extracts were combined. Total polyphenol content was estimated using the Folin-Ciocalteu method (22), and the results were expressed in mg of gallic acid equivalents (GAE) per g sample (dry mass basis) with a mass of 0–200 mg of gallic acid according to the calibration curve:
y=0.0031x+0.009; R2=0.9965 /4/
Microencapsulation and characterization of microcapsules
The three different types of derivatized starch (phosphorylated, acetylated and double esterified starch) were used for microencapsulation of red sorghum polyphenols by spray drying (SD-Basic spray dryer; LabPlant, Huddersfield, UK). Emulsions of 10 g of starch esterified by extrusion, 60 mL of the polyphenolic extract and 30 mL of distilled water were prepared. The internal phase was added at 30 °C to form the emulsion immediately prior to drying. Emulsions were homogenized with an Ultra Turrax T-25-SI homogenizer (IKA Works, Wilmington, NC, USA) at 14 000 rpm for 5 min. The dispersion was maintained under constant stirring with a magnet bar during drying. Drying conditions were as follows: inlet air temperature (150±1) °C, outlet air temperature (80±5) °C, nozzle diameter 0.5 mm, and a liquid flow rate 10 mL/min. The airflow equipment was set at 70 m3/h. Dried powder microcapsules were collected from the base of the cyclone for subsequent characterization.
Efficiency and yield of encapsulation
Encapsulation efficiency (EE) and encapsulation yield (EY) were quantified by determining the concentration of superficial and total polyphenols (23). For determination of the concentration of superficial polyphenols, a sample (200 mg) of microcapsules was treated with 2 mL of ethanol and methanol (50:50); these dispersions were stirred in a vortex (Genius 3; IKA, Wilmington, NC, USA) for 1 min and then filtered (0.45 μm pore). For determination of total polyphenols, microcapsules (200 mg) were dispersed in 2 mL of methanol, acetic acid and water (50:8:42 V/V/V), stirred using a vortex mixer (Genius 3; IKA) for 1 min, ultrasonicated in a 20-kHz Branson ultrasonic bath (Dietzenbach, Germany) twice for 20 min, and then centrifuged at 112 000×g for 5 min. Finally, the residues of both total and superficial polyphenols were quantified according to the Folin-Ciocalteu method (22) as mentioned above.
EE and EY were determined according to the following equations:
EE=(w(total polyphenols–surface polyphenols))/ w(total polyphenols)·100 /5/
and
EY=w(total polyphenols in microcapsules)/
w(total polyphenols in emulsion)·100 /6/
Differential scanning calorimetry
Differential scanning calorimetry (DSC) was used to investigate the thermal properties of microcapsules (loaded with sorghum polyphenols) of phosphorylated, acetylated, double esterified starch and Capsul® starch employing a DSC instrument (Mettler Toledo model 821; Schwerzenbach, Switzerland) calibrated with indium. Each sample (7 mg) was heated on an aluminium pan at a rate of 10 °C/min at between 25 and 210 °C.
Scanning electron microscopy
The morphological characteristics of the microcapsules were evaluated using SEM (Philips XL30; Morrisville, NC, USA) at an acceleration voltage of 3 kV. Dried microcapsule samples were previously sprinkled on a double-sided sticky tape placed on aluminium stubs and covered with thin graphite film.
Accelerated storage stability test
Microcapsules loaded with red sorghum phenolic compounds were stored at 60 °C in an oven with controlled temperature and in the absence of light for 35 days. Samples of 200 mg of microcapsules (phosphorylated, acetylated and double esterified starch) and Capsul® (as control wall material) were transferred into 100 mm×150 mm clear glass vials. Determination of total polyphenols was performed to evaluate changes during storage. Triplicate vials were removed every 7 days.
Statistical analysis
All experiments were performed in triplicate. The differences between mean values of the treatments were determined using analysis of variance (ANOVA) and the paired Tukey’s test (p<0.05) with IBM SPSS v. 20 statistical software (24).
ACKNOWLEDGEMENTS
ACKNOWLEDGEMENTS
We would like to thank CONACYT, Mexico and Dr Víctor Pecina for the donation of sorghum. We thank Verónica Flores Casamayor, MSc, José Juan Véles Medina, MSc, Jose Eleazar Urbina Alvarez BSc and Carlos Alberto Ávila Herrera, BSc, from Cinvestav-Querétaro for their technical support.